With the rapid advancements in battery technology and the increasing demand for electric vehicles, choosing the right battery pack for business purposes has become more crucial than ever. Recent trends indicate a surge in the market, driven by innovations like solid-state and graphene-based batteries. This article delves into the essential factors to consider, providing professional buyers with valuable insights for making informed decisions.
Table of Contents:
– Market Overview: Battery Pack Industry
– In-Depth Analysis of the Battery Pack Market
– Key Factors When Selecting a Battery Pack
– Emerging Technologies in Battery Packs
– Regulatory Compliance and Environmental Impact
– Conclusion
Market Overview: Battery Pack Industry
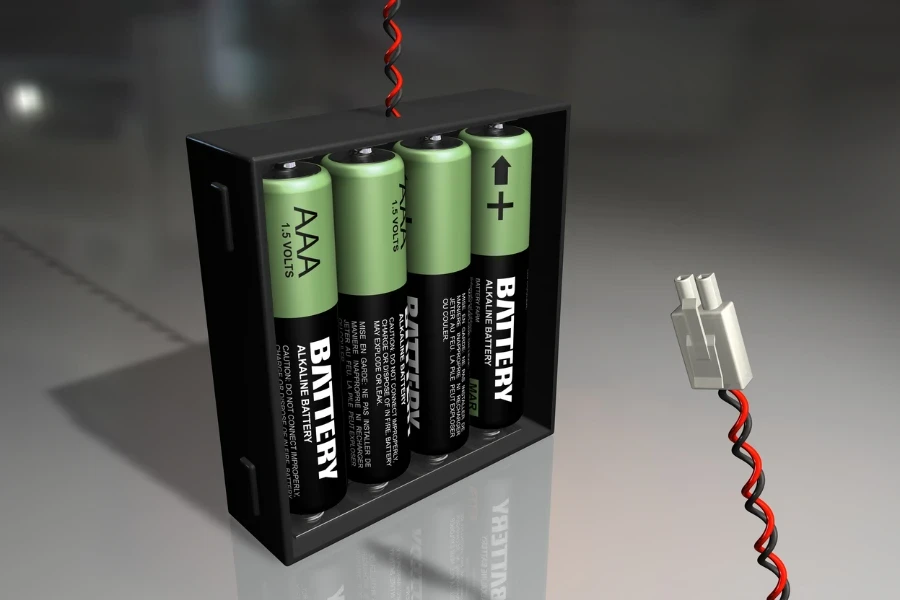
The global battery pack market has seen significant growth, driven by the increasing adoption of electric vehicles (EVs) and advancements in battery technology. Recently, the market was valued at USD 66.98 billion and is projected to reach USD 199.90 billion by 2030, with a compound annual growth rate (CAGR) of 16.90%. This growth is due to the rising demand for zero-emission vehicles and continuous improvements in battery energy density and charging speed.
Asia-Pacific holds the largest market share, mainly due to China’s aggressive industrial policies, substantial investments in battery technology, and a large consumer base. Major battery manufacturers and local production facilities also support the region’s dominance. The Americas and Europe are also experiencing significant growth, driven by government incentives and the expansion of electromobility. For example, the U.S. Department of Energy has allocated USD 2.8 billion to expand domestic battery manufacturing, highlighting the region’s commitment to clean energy.
Additionally, the market for e-bike battery packs is expected to grow from USD 10.76 billion in 2024 to USD 24.19 billion by 2030, at a CAGR of 14.3%. This growth is fueled by the increasing demand for eco-friendly transportation, advances in battery technology, and government incentives for electric vehicles.
In-Depth Analysis of the Battery Pack Market
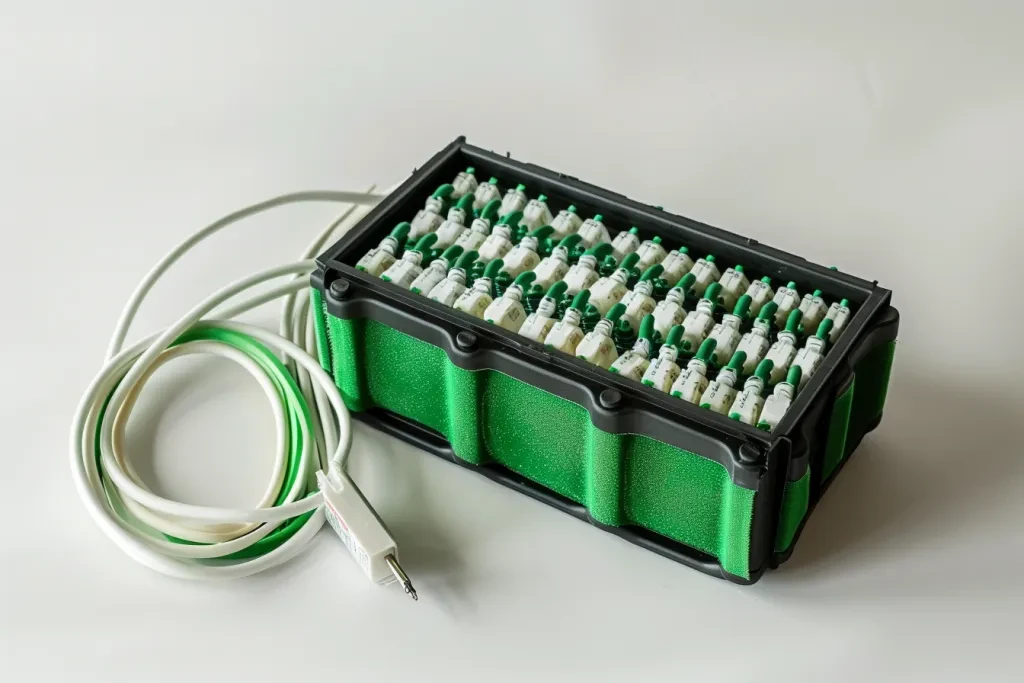
The battery pack market is defined by key performance benchmarks, market share dynamics, and recent innovations. One primary goal of cell-to-pack battery technology is to reduce costs and increase volumetric density. By integrating battery cells directly into a pack without intermediary modules, this technology enhances energy density and efficiency. The market is expected to grow from USD 6.27 billion recently to USD 30.72 billion by 2030, with a CAGR of 25.47%.
Economic factors like raw material price volatility and the need for continuous technological advancements pose challenges. However, the rise in battery materials processing facilities and ongoing innovations are expected to create lucrative opportunities. For instance, solid-state and sodium-ion batteries are gaining traction due to their higher energy density, faster charging capabilities, and improved safety features.
Consumer behavior is shifting towards sustainable transportation options, with a growing preference for electric vehicles and e-bikes. Seasonal demand patterns also impact the market, with higher sales during favorable weather conditions for outdoor activities. Distribution channels are evolving, with a focus on direct-to-consumer sales and strategic partnerships with technology developers.
Environmental regulations play a crucial role in shaping the market, with governments implementing policies to reduce carbon emissions and promote clean energy. For example, the European Union’s stringent CO2 emission standards are driving the adoption of electric vehicles and battery packs. Additionally, concerns about battery disposal and recycling are being addressed through innovations in recycling methods and the development of second-life applications for EV batteries.
Brand positioning and differentiation are critical for market players to gain a competitive edge. Leading vendors like CATL, LG Energy Solution, and Panasonic are investing in research and development to enhance product offerings and maintain market leadership. The FPNV Positioning Matrix is a valuable tool for evaluating vendors based on business strategy and product satisfaction, helping companies make informed decisions and devise effective strategies.
Key Factors When Selecting a Battery Pack
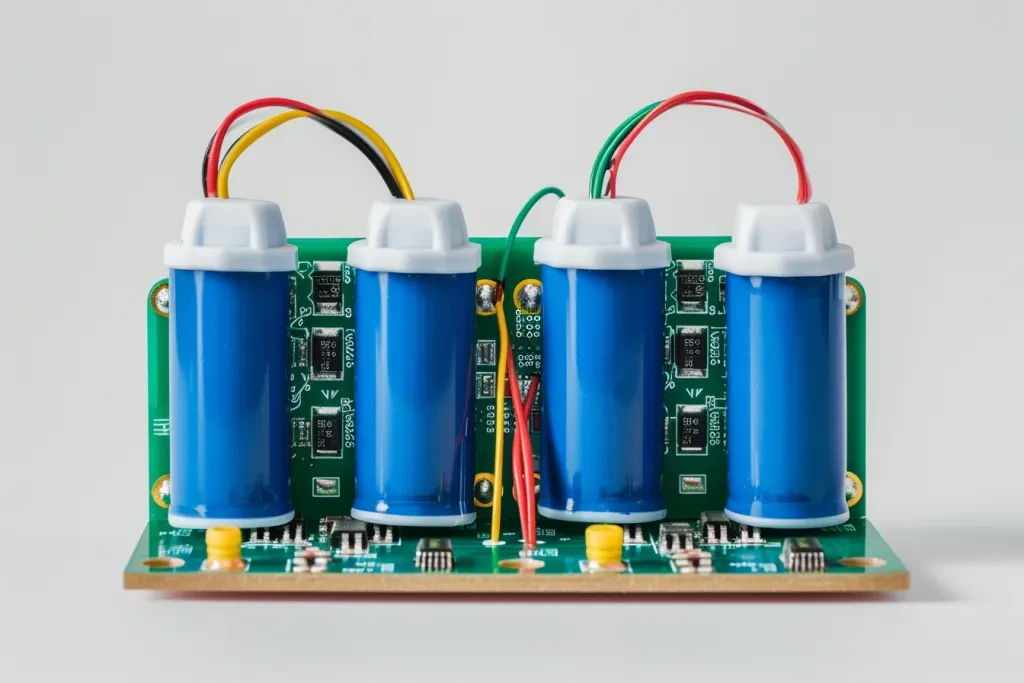
Capacity and Energy Density
When choosing a battery pack, consider its capacity, typically measured in milliampere-hours (mAh) or watt-hours (Wh). Capacity indicates how much energy the battery can store and deliver. A higher capacity battery provides longer usage times between charges, which is essential for devices like laptops, smartphones, and electric vehicles. Energy density, expressed as Wh/kg, shows how much energy a battery stores relative to its weight. High energy density batteries are ideal for portable electronics where weight matters.
Understanding energy density also helps assess battery efficiency. For example, lithium-ion batteries generally offer higher energy densities than nickel-metal hydride (NiMH) batteries, making them popular for high-performance applications. Ensure the battery pack’s capacity and energy density meet your device’s requirements.
Cycle Life and Durability
Cycle life refers to the number of complete charge and discharge cycles a battery can undergo before its capacity significantly degrades. This is crucial for applications needing long-term reliability. For instance, lithium-ion batteries in consumer electronics typically offer a cycle life of 300 to 500 cycles, with some modern batteries providing up to 1000 or more cycles.
Durability is also important, especially for devices exposed to harsh environments or frequent physical stress. Batteries with robust casings and advanced internal protection mechanisms are preferable. For example, ruggedized battery packs used in industrial settings often feature enhanced durability to withstand extreme temperatures, vibrations, and impacts. Choosing a battery pack with high cycle life and durability ensures longevity and reliability.
Charging Speed and Efficiency
Charging speed is vital, especially for users needing quick turnaround times. Fast charging technologies, like Qualcomm Quick Charge or USB Power Delivery (PD), can significantly reduce charging times. For example, a USB PD-supported battery pack can charge a compatible device up to 50% in just 30 minutes. Charging efficiency affects overall energy consumption and thermal management.
A battery pack’s charge acceptance rate indicates its efficiency during charging. A higher rate means the battery can accept more power in less time, reducing charging duration. Efficient charging also reduces heat generation, prolonging the battery’s lifespan. Therefore, consider both fast charging protocols and charging efficiency when selecting a battery pack.
Safety Standards and Certifications
Safety is non-negotiable in battery pack selection. Compliance with international safety standards and certifications ensures the battery meets stringent safety and performance criteria. Common certifications include UL (Underwriters Laboratories), CE (Conformité Européenne), and RoHS (Restriction of Hazardous Substances). These certifications guarantee rigorous testing for safety, reliability, and environmental compliance.
For instance, UL-certified battery packs are tested for hazards like overcharging, short-circuiting, and thermal runaway. RoHS compliance ensures the battery pack is free from harmful substances like lead and mercury, making it environmentally friendly. Verify that the battery pack has the necessary safety certifications for safe operation and regulatory compliance.
Compatibility and Integration
Compatibility with the intended device and ease of integration are critical. The battery pack should match the device’s voltage, current, and connector specifications. Using a battery pack with an incorrect voltage rating can damage the device or reduce its performance. The physical dimensions and form factor should also fit seamlessly into the device’s design.
Integration involves physical, electrical, and communication compatibility. Modern battery packs often feature smart battery management systems (BMS) that communicate with the device to optimize performance and safety. Ensure the battery pack’s BMS is compatible with the device’s power management system for efficient operation. Thorough compatibility checks and integration assessments are necessary when selecting a battery pack.
Emerging Technologies in Battery Packs
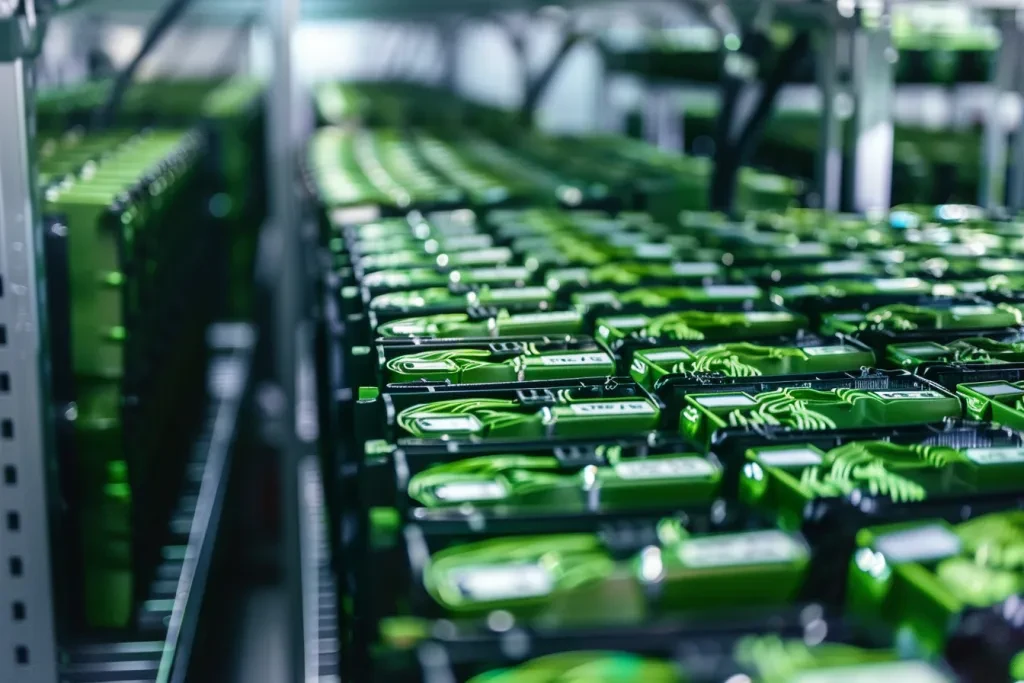
Solid-State Batteries
Solid-state batteries represent a significant advancement, offering higher energy densities, improved safety, and longer cycle lives compared to traditional lithium-ion batteries. These batteries use a solid electrolyte, reducing the risk of leakage and thermal runaway. For example, a solid-state battery can achieve energy densities of up to 500 Wh/kg, compared to 250 Wh/kg for conventional lithium-ion batteries.
The improved safety profile makes solid-state batteries ideal for applications where safety is paramount, such as electric vehicles and aerospace. Their longer cycle life can reduce the total cost of ownership by extending battery replacement intervals. As research and development in solid-state technology advance, these batteries are expected to become more prevalent in consumer electronics and other high-demand applications.
Graphene-Based Batteries
Graphene, a single layer of carbon atoms arranged in a hexagonal lattice, has emerged as a promising material for enhancing battery performance. Graphene-based batteries offer higher conductivity, faster charging times, and greater durability. For instance, a graphene-enhanced lithium-ion battery can charge up to five times faster than a conventional battery, significantly reducing downtime.
Graphene’s high mechanical strength and flexibility make it excellent for developing flexible and wearable electronics. Incorporating graphene into battery electrodes can improve thermal management, reducing overheating risks and extending battery lifespan. As production and integration of graphene become more cost-effective, graphene-based batteries are expected to revolutionize the battery industry.
Nanotechnology in Batteries
Nanotechnology involves manipulating materials at the atomic and molecular levels to enhance their properties. In batteries, nanotechnology can improve energy storage capacity, charge-discharge rates, and overall efficiency. For example, using nanostructured materials in battery electrodes can increase surface area, allowing more active sites for electrochemical reactions and higher energy storage.
Nanocoatings can protect battery components from degradation, enhancing durability and cycle life. Nanotechnology can also enable new battery chemistries, such as lithium-sulfur and lithium-air batteries, which offer higher energy densities than traditional lithium-ion batteries. Advancements in nanotechnology hold great potential for creating more efficient and long-lasting energy storage solutions.
Regulatory Compliance and Environmental Impact
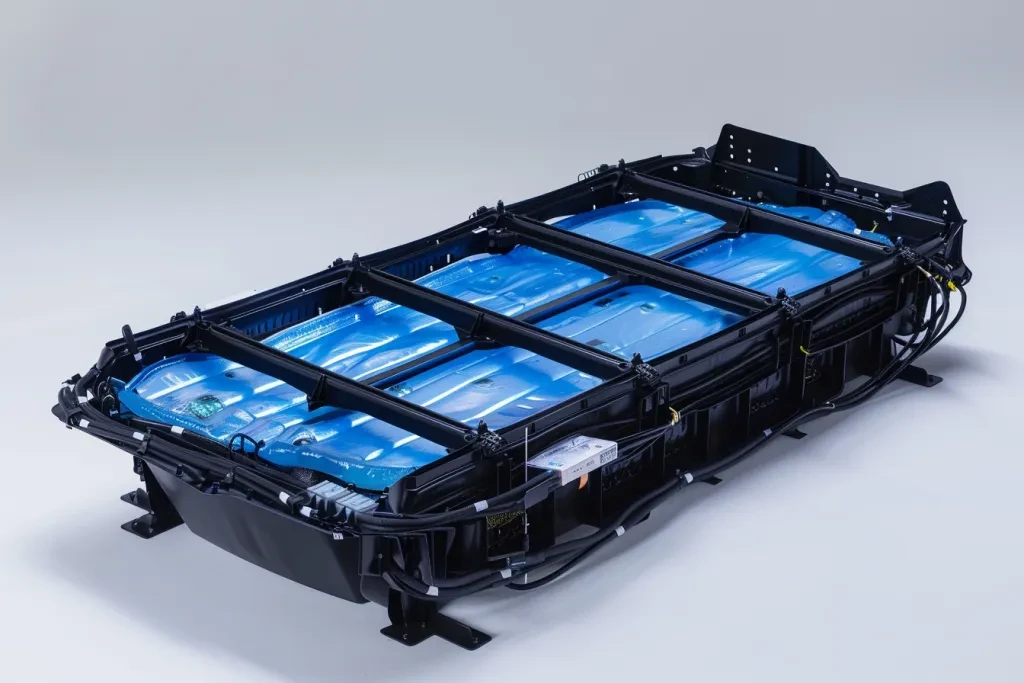
Compliance with Environmental Regulations
Battery packs must comply with environmental regulations to ensure they do not pose risks to the environment. Regulations like the Restriction of Hazardous Substances (RoHS) and the Waste Electrical and Electronic Equipment (WEEE) directive in the European Union mandate proper disposal and recycling of batteries. Compliance ensures the battery pack is free from harmful substances and can be safely recycled at the end of its lifecycle.
For example, RoHS compliance indicates the battery pack is free from restricted hazardous materials, making it safer for users and the environment. Adhering to WEEE directives ensures manufacturers take responsibility for battery disposal and recycling, reducing environmental impact. Verifying that a battery pack complies with relevant environmental regulations is essential for sustainable and responsible usage.
Recycling and Disposal
Proper recycling and disposal of battery packs minimize environmental impact and recover valuable materials. Many battery packs contain materials like lithium, cobalt, and nickel, which can be recovered and reused in new batteries. Recycling conserves natural resources and reduces environmental hazards, such as soil and water contamination.
Manufacturers often provide guidelines for safe disposal and recycling of battery packs, and many countries have established recycling programs. For example, in the United States, the Call2Recycle program offers convenient drop-off locations for recycling batteries. Ensuring proper disposal and recycling is critical for environmental stewardship and regulatory compliance.
Impact on Carbon Footprint
The production, usage, and disposal of battery packs contribute to the overall carbon footprint of electronic devices. Selecting battery packs with lower environmental impact can help reduce this footprint. For instance, battery packs manufactured using renewable energy sources or sustainable materials have a lower carbon footprint than those produced using conventional methods.
Battery packs with longer lifespans and higher energy efficiencies reduce the need for frequent replacements, further minimizing environmental impact. Evaluating the carbon footprint of battery packs involves considering the entire lifecycle, from raw material extraction to manufacturing, usage, and disposal. Choosing battery packs with lower carbon footprints contributes to environmental sustainability and reduces overall impact.
Conclusion
In summary, selecting the right battery pack involves considering capacity, cycle life, charging speed, safety standards, and compatibility. Emerging technologies like solid-state batteries, graphene-based batteries, and nanotechnology are set to revolutionize the industry, offering enhanced performance and safety. Regulatory compliance and environmental impact are also critical considerations for sustainable and responsible usage. By thoroughly evaluating these factors, buyers can make informed decisions and choose battery packs that meet their needs and contribute to overall efficiency and sustainability.